Timisoara_Med 2022, 2022(2), 2; doi:10.35995/tmj20220202
Original Paper
Cell-Permeable Succinate Improves Platelet Respiration in Patients Undergoing Cardiopulmonary Bypass: A Pilot Study
1
Institute of Cardiovascular Diseases, “Victor Babeș” University of Medicine and Pharmacy, Timișoara, 2 Eftimie Murgu Sq., 300041 Timișoara, Romania; adimerce@yahoo.com (A.P.M.); petrescu_lucian@yahoo.com (L.P.)
2
Center for Translational Research and Systems Medicine, “Victor Babeș” University of Medicine and Pharmacy, Timișoara, 2 Eftimie Murgu Sq., 300041 Timișoara, Romania; lungu.anca@umft.ro (A.M.B.); darius_buriman@yahoo.com (D.G.B.); lascu.ana@umft.ro (A.L.)
3
Department III—Functional Sciences—Pathophysiology, “Victor Babeș” University of Medicine and Pharmacy, Timișoara, 2 Eftimie Murgu Sq., 300041 Timișoara, Romania
4
Department VII—Internal Medicine—Diabetes, Nutrition and Metabolic Diseases, “Victor Babeș” University of Medicine and Pharmacy, Timișoara, 2 Eftimie Murgu Sq., 300041 Timișoara, Romania; avram.vlad@umft.ro
5
Department VI—Cardiology—Cardiovascular Surgery, “Victor Babeș” University of Medicine and Pharmacy, Timișoara, 2 Eftimie Murgu Sq., 300041 Timișoara, Romania; horea.feier@gmail.com
6
Mitochondrial Medicine, Department of Clinical Sciences, Faculty of Medicine, Lund University, BMC A13, 221 84 Lund, Sweden
7
Abliva AB, Medicon Village, 223 81 Lund, Sweden
8
Department IX—Surgery—Surgical Semiology I, “Victor Babeș” University of Medicine and Pharmacy, Timișoara, 2 Eftimie Murgu Sq., 300041 Timișoara, Romania; octavian.cretu@umft.ro
9
Center for Hepato-Biliary and Pancreatic Surgery, “Victor Babeș” University of Medicine and Pharmacy, Timișoara, 2 Eftimie Murgu Sq., 300041 Timișoara, Romania
*
Correspondence: daninamuntean@umft.ro (D.M.M.); eskil.elmer@med.lu.se (E.E.)
†
These authors contributed equally to this work.
How to cite: Merce, A.P.; Bînă, A.M.; Avram, V.F.; Buriman, D.G.; Lascu, A.; Feier, H.B.; Petrescu, L.; Muntean, D.M.; Elmér, E.; Crețu, O.M. Cell-Permeable Succinate Improves Platelet Respiration in Patients Undergoing Cardiopulmonary Bypass: A Pilot Study. Timisoara Med. 2022, 2022 (2), 2; doi:10.35995/tmj20220202.
Received: 24 July 2022 / Accepted: 4 August 2022 / Published: 16 August 2022
Abstract
:Open-heart surgery with cardiopulmonary bypass (CPB) remains the standard approach for complex cardiac pathologies, such as advanced coronary heart disease and severe valvular defects. Platelet dysfunction has been widely reported, with both structural and functional changes being elicited by the CPB circuit. Succinate is a mitochondrial substrate that is metabolized through complex II (CII) but is impermeable to cellular membranes when given exogenously. Cell-permeable succinates are novel prodrugs developed to support mitochondrial electron transport (ET) and prevent energy depletion in various pathologies. The aim of the present pilot study was to investigate the role of NV118 (diacetoxymethyl succinate), a cell-permeable succinate, on platelet respiration in a pilot group of patients undergoing CPB. Blood samples (20 mL) were collected from participants before (prior to heparin administration) and after CPB (within 10 min after protamine sulphate administration). Platelets were isolated through a two-step centrifugation protocol. Mitochondrial respiration was analyzed by means of high-resolution respirometry in the presence of NV118 or its solvent (DMSO). The main respiratory parameters recorded were as follows: ROUTINE respiration, LEAK respiration, and maximal uncoupled respiration for both CI and CII (ET capacity) and for CII solely after CI inhibition (ET CII capacity). Here, we report that NV118 elicited a global increase in platelet respiration both pre- and post-CPB. In conclusion, NV118, a cell-permeable succinate, improved platelet bioenergetics in the setting of cardiopulmonary bypass. Whether the compound can support platelet function and/or provide organ protection at the mitochondrial level during CPB are clearly worthy and important areas for future investigation.
Keywords:
cardiopulmonary bypass; platelets; mitochondrial respiration; high-resolution respirometry; cell-permeable succinate1. Introduction
One of the most important medical advances of the 20th century was the development of cardiopulmonary bypass (CPB) which paved the way to open-heart surgery [1]. While being an irreplaceable part of cardiothoracic surgery, it is well established that extracorporeal circulation systems are associated with the activation of inflammatory and oxidative stress responses [2,3]. Moreover, the exposure of blood to the synthetic surfaces of the heart–lung machine as well as to non-physiological shear stress due to mechanical pumping are responsible for the perioperative hemostatic abnormalities; both bleeding and thrombotic events were reported as major causes of morbidity and mortality in these patients [4,5]. In particular, the CPB circuit determines several structural and functional platelets abnormalities. The activation of platelets that remain in circulation by the bypass circuit will render them hypo-responsive to stimulation with various agonists, which may impair their hemostatic properties [6].
The last decade has witnessed an increased interest for another pathomechanism in the setting of open-heart surgery, namely mitochondrial dysfunction. During CPB, cardiac mitochondrial dysfunction results from systemic inflammation, surgical trauma, and ischemia/reperfusion injury, and in turn, impairs postoperative myocardial contractility and predisposes one to arrhythmias [7]. Not only cardiac but also cerebral mitochondrial dysfunction was reported to occur during CPB. A recent study performed in piglets subjected to 4 h of CPB showed that, even in the absence of local markers of ischemia, CPB was associated with decreased mitochondrial respiration and increased mitochondrial ROS formation in brain cortex homogenates [8].There is one pioneering study in the literature that assessed the mitochondrial respiration of intact platelets isolated from adult patients before and after CPB using the extracellular flux analyzer Seahorse XF24; these authors found neither changes in respiratory parameters nor an association between platelet respiration and aggregation/postoperative bleeding [9]. Of note, the latter observation has been also recapitulated in chronic conditions by the group of Cezar Watala; in a recent elegant study performed in the experimental model of type 1 diabetes, they reported that elevated platelet mitochondria respiration occurred later in the evolution of the disease and was not related to the earlier observed changes in platelet activation/reactivity [10].
However, the intraoperative impairment of platelets due to blood exposure to the CPB circuit occurs in cardiac surgery dependent on its duration, and whether their damage can be rescued has not been investigated so far. Nowadays, several pharmacological strategies for mitochondrial protection or recovery after injury are currently under investigation. Cell-permeable succinates are prodrugs able to pass over the cellular membrane, release succinate in the cytosol, and enhance mitochondrial ATP production. They have been developed to bypass mitochondrial complex I defects [11] and have been reported to support electron transport in various pathologies associated with complex I dysfunction/inhibition [12,13].
The present pilot study aimed to assess whether a first-generation cell-permeable succinate, NV118 (diacetoxymethyl succinate), is able to modulate platelets bioenergetics in patients undergoing cardiopulmonary bypass. Here, we report that NV118 elicited a global improvement in the respiratory function of intact platelets isolated both prior to and after cardiopulmonary bypass.
2. Materials and Methods
2.1. Study Population
The study was conducted in accordance with the statements of the Declaration of Helsinki, and the protocol was approved by the Committee of Research Ethics of “Victor Babeș” University of Medicine and Pharmacy, Timișoara, Romania (no. 04/28.02.2020 and no. 04p/17/12/2020). The study protocol was explained, and all participants provided written informed consent.
Thirteen patients (four M, nine F) diagnosed with multivessel coronary artery disease, valvular pathology, or both were admitted for open-heart surgery with CPB in a single center (Institute for Cardiovascular Diseases of Timișoara) and included in the study. The exclusion criteria were: heart failure > NYHA III and ejection fraction < 40%, age < 18 or > 80 years, platelet count < 100.000/mm3, hemoglobin < 8 g/dL, congenital or acquired platelets disorders, congenital coagulation disorders, disseminated intravascular coagulation, and surgical emergencies. The clinical and laboratory characteristics of the study group are presented in Table 1.
Regarding the type of surgery, 3 (23%) patients underwent aortic valve replacement, 1 (7.69%) patient had mitral valve replacement, 5 (38.4%) patients underwent aorto-coronary bypass graft, and 4 (30.7%) patients had aortic valve replacement and aorto-coronary bypass surgery. The mean duration of CBP was 90 min. All patients presented preoperative hypertension as a comorbidity for which antihypertensive medication (ACE inhibitors/sartans ± calcium channel blockers) was administered.
2.2. Platelet Isolation
Twenty ml of peripheral blood was collected before (prior to heparin administration) and after the CPB (within 10 min after protamine sulphate administration) using K2(EDTA) as an anticoagulant. Blood samples were prepared and analyzed within 1–4 h after collection. The platelet suspension dissolved in 1 mL of homologous plasma was obtained through two-step centrifugation at 22 °C (10 min at 500× g, 5 min at 4600× g) according to a protocol adapted from ref. [14] and further used for high-resolution respirometry studies.
2.3. High-Resolution Respirometry
The mitochondrial respiration of isolated intact (non-permeabilized) platelets was assessed at 370C through high-resolution respirometry (HRR) using an oxygraph-2k (Oroboros Instr., Innsbruck, AT, USA). Platelets (200 million/chamber) were suspended in a mitochondrial respiration medium (MiR05): sucrose 110 mM, HEPES 20 mM, taurine 20 mM, K-lactobionate 60 mM, MgCl2 23 mM, KH2PO4 10 mM, EGTA 0.5 mM, BSA 1 g/L, and pH 7.1, as described in refs. [14,15]. Prior to each experiment, calibration at air saturation was performed. A Substrate–Uncoupler–Inhibitor–Titration (SUIT) protocol was adapted from ref. [16] to measure complex I (CI) and complex II (CII)-dependent respiration in intact platelets. In brief, the platelet oxygen consumption rate (OCR) was allowed to reach a steady state corresponding to the ROUTINE respiration (respiration in the physiological coupling state). A cell-permeable succinate NV118 (500 µM) or an equivalent volume of dimethyl sulfoxide (DMSO) as a control was added in the 4xygraphy chambers. ATP-synthase was inhibited using oligomycin (1 μg/mL) in order to assess LEAK respiration (the non-phosphorylating respiration), after which, a stepwise titration of FCCP (a protonophore) was performed in order to assess the ET CAPACITY (maximal uncoupled respiration as an indicator of the maximal activity of the electron transport system—ETS). Last but not least, in order to investigate the direct effects of NV118 on complex II-supported respiration, complex I was inhibited with rotenone (2 µM), allowing the measurement of ETII capacity (the residual succinate-supported respiration). In the end, complex III was inhibited with antimycin A (1 µg/mL), allowing the estimation of residual oxygen consumption (ROX). The OCR was expressed in pmol × s−1 × 2 × 10−8 platelets, and all the values were ROX-corrected.
2.4. Chemicals
All chemicals were obtained from Sigma-Aldrich-Merck. The cell-permeable succinate was generously provided by Abliva AB (Lund, Sweden) and is available in the MitoKit-CII (Oroboros Instr., AT).
2.5. Data Analysis
DatLab software (Oroboros Instr.) was used to record the oxygen concentration and oxygen consumption rate (flux) displayed in real time and also for data analysis. Mitochondrial respiration was also corrected for oxygen flux due to instrumental background.
Statistical analysis was performed using GraphPad software (version 9.0). All data are expressed as mean ± SEM of ROX corrected respiration.
3. Results
DMSO was used as the solvent for NV118, and its effect on ROUTINE respiration was firstly assessed. As depicted in Figure 1, no differences in platelet physiological OCR were found prior to (8.98 ± 0.36 vs. 8.96 ± 0.36 pmol × s−1 × 2 × 10−8 cells—Figure 1A) or after (11.50 ± 0.58 vs. 11.51 ± 0.56 pmol × s−1 × 2 × 10−8 cells—Figure 1B) cardiopulmonary bypass in the presence of DMSO in the oxygraph chambers.
Furthermore, the effect of NV118 (as compared to DMSO) on intact platelets, isolated both prior to and after CPB, was assessed by recording the above-mentioned respiratory parameters: ROUTINE respiration, LEAK respiration, ET capacity, and ETII.
3.1. Cell-Permeable Succinate Improved Mitochondrial Respiration Prior to CPB
As depicted in Figure 2, the prodrug NV118 elicited a global increase in the respiratory parameters (pmol × s−1 × 2 × 10−8 cells) in isolated platelets harvested before the surgery. ROUTINE respiration (Figure 2A) significantly increased from 8.96 ± 0.36 to 14.77 ± 0.54 (p < 0.0001), LEAK respiration (Figure 2B) slightly increased from 2.54 ± 0.57 to 4.99 ± 0.88 (p < 0.05), whereas the maximal uncoupled respiration dependent on both mitochondrial complexes CI+CII (Figure 2C) almost doubled (from 14.06 ± 1.24 to 26.31 ± 1.95, p < 0.0001). Finally, the residual succinate-supported respiration was measured after rotenone addition to inhibit CI. As shown in Figure 2D, the ET capacity dependent on CII significantly increased from 1.15 ± 0.23 to 5.36 ± 0.41 (p < 0.0001), confirming the beneficial effect of NV118, the CII substrate.
3.2. Cell-Permeable Succinate Improved Mitochondrial Respiration after CPB
Platelets isolated after CPB were exposed to NV118 vs. DMSO, with a comparable increase for almost all respiratory parameters (pmol × s−1 × 2 × 10−8 cells), as previously described prior to CPB. Thus, ROUTINE respiration (Figure 3A) significantly increased from to 11.51 ± 0.58 to 16.77 ± 0.99 (p < 0.001), while LEAK respiration (Figure 3B) slightly increased from 2.97 ± 0.42 to 4.71 ± 0.61 (p < 0.05). The maximal uncoupled respiration dependent on both mitochondrial complexes CI + CII (Figure 3C) also showed higher values (from 19.52 ± 1.42 to 27.42 ± 1.97, p < 0.01), and the ETII capacity (Figure 3D) significantly increased from 1.38 ± 0.28 to 6.44 ± 0.91 (p < 0.0001), as recorded prior to CPB.
A representative trace of the high-resolution respirometry of intact platelets isolated after CPB in the presence of NV118 (green) is depicted in Figure 4.
4. Discussion
The main finding of this pilot study is the improvement (via NV118) of mitochondrial bioenergetics in intact platelets isolated from patients prior to and after cardiopulmonary bypass. To our knowledge, this is the first study which investigated the effect of cell-permeable succinate on platelets exposed to extracorporeal circulation. Here, we report a significant increase in all respiratory parameters, in the presence of NV118, in isolated platelets, regardless of the moment of their harvesting.
In particular, routine respiration is dependent on the existent mitochondrial matrix succinate that platelets retain while ex vivo. Since the addition of a membrane-permeable succinate increased oxygen consumption both pre- and post-CPB, this indicates that platelet oxidative metabolism is limited by the concentration of succinate as an energetic substrate. The addition of a cell-permeable succinate would correct this in any condition. From a translational perspective, this observation may have significant clinical implications. For example, the administration of permeable succinates in extracorporeal circulation will correct the substrate deficit and improve platelet bioenergetics.
As previously reported by the Gary Fiskum group in their pioneering work that analyzed platelet respiration in the setting of CPB using the Seahorse extracellular flux analyzer [9], we did not find significant differences in platelet respiration prior to or after CPB using the HRR technique in this pilot study (data not shown). The mean duration of CBP was 1.5 h in this group. We cannot exclude the possibility that longer periods of extracorporeal circulation and/or the presence of metabolic comorbidities (reported to be associated with mitochondrial dysfunction) in patients undergoing open-heart surgery might elicit platelet mitochondrial respiratory dysfunction.
A wide range of pharmacological approaches have been studied to counteract the pathomechanisms responsible for the potential deleterious effects of CPB, namely to mitigate systemic inflammation [17], oxidative stress [18], and ischemia/reperfusion injury lesions [19] and provide metabolic support [20].
In recent years, the use of peripheral platelets as an easy obtainable source of viable mitochondria has been brought to light, which allows the analysis of mitochondrial respiratory dys/function [21] in either acute pathologies such as sepsis [22], cardiac arrest [23], acute leukemia [24], and preeclampsia [25] or chronic diseases such as diabetes [26], obesity [27], and asthma [28]. Additionally, the past few decades witnessed an increasing interest in the development of drugs capable of supporting mitochondrial function, and cell-permeable succinates have emerged as such compounds. Succinate is the substrate of succinate dehydrogenase, which is complex II (embedded in the inner mitochondrial membrane), being crucial for oxidative phosphorylation as it couples the tricarboxylic cycle with the electron transport system activity. Succinate causes the ETS to run sequentially from complex II (i.e., it bypasses CI) all the way down to complex IV, where oxygen is converted to water [29]. Thus, it is particularly useful in pathological conditions associated with dysfunctional CI either due to genetic mutations or damage due to increased oxidative stress or drug toxicity (more frequent in practice). However, succinate is not cell-permeable (being an anion in living organisms), so it has limited intracellular uptake when exogenously administered. To date, cell-permeable succinate prodrugs have emerged as alternative fuel sources in order to prevent energetic dysfunction in various pathological conditions. Accordingly, succinate prodrugs have been reported to improve mitochondrial respiration in cellular models of drug toxicity [12,13,30], peripheral blood mononuclear cells from acute poisoning with carbon monoxide [31], and fibroblasts cultured from a patient with Leigh syndrome (mitochondrial disease with CI defect) [32].
Whether cardiac and cerebral mitochondrial dysfunction reported to occur in some of the patients undergoing CPB can be alleviated by cell-permeable succinates awaits future investigation.
We acknowledge the small number of subjects investigated, and thus, the limited statistical power as an important limitation of this pilot study.
5. Conclusions
NV118, a cell-permeable succinate prodrug, improved platelet bioenergetics in the setting of cardiopulmonary bypass. Whether this effect can support platelet function during CPB is yet another area of enquiry for future investigation. This finding may also be a voice in the discussion on new pharmacological approaches of organ protection during cardiac surgery.
Author Contributions
Conceptualization, A.P.M., A.M.B., D.M.M., and E.E.; methodology, A.P.M., A.M.B., and V.F.A.; formal analysis, D.M.M., L.P., E.E., and OM.C.; investigation, A.P.M., A.M.B., A.L., D.G.B., V.F.A., and H.B.F.; data curation, A.P.M., A.M.B., D.G.B., and D.M.M.; writing—original draft preparation, A.P.M., A.M.B., and D.M.M.; writing—review and editing, A.P.M., A.M.B., A.L., D.G.B., and V.F.A.; visualization, A.P.M., A.M.B., A.L., D.G.B., V.F.A., H.B.F., L.P., D.M.M., E.E., and O.M.C.; supervision, D.M.M., E.E., and O.M.C.; project administration, O.M.C.; funding acquisition, O.M.C. All authors contributed to the article and approved the submitted version.
Institutional Review Board Statement
The study protocol and informed consent were approved by the Committee of Research Ethics of “Victor Babeș” University of Medicine and Pharmacy, Timișoara, Romania (no. 04/28.02.2020 and 04p/17/12/2020).
Acknowledgments
We are grateful to Abliva AB for generously providing the NV118 compound. We acknowledge the expert technical assistance of Andreea Anechitei.
Conflicts of Interest
E.E. has or has had a salary from and/or equity interest in Abliva AB (previously named NeuroVive Pharmaceutical AB), a company active in the field of mitochondrial medicine. E.E. has filed patent applications for the use of succinate prodrugs for the treatment of lactic acidosis or drug-induced side effects due to the complex I-related impairment of mitochondrial oxidative phosphorylation (WO/2015/155238) and protected carboxylic acid-based metabolites for the treatment of mitochondrial disorders (WO/2017/060400, WO/2017/060418, WO/2017/060422). This does not alter the adherence to manuscript policies on sharing data and materials. Abliva AB had no role in the study design, the data collection and analysis, or the preparation of the manuscript. The remaining authors declare that they have no known competing financial interests or personal relationships that could have appeared to influence the work reported in this paper.
References
- Holman, W.L.; Timpa, J.; Kirklin, J.K. Origins and Evolution of Extracorporeal Circulation: JACC Historical Breakthroughs in Perspective. J. Am. Coll. Cardiol. 2022, 79, 1606–1622. [Google Scholar] [CrossRef] [PubMed]
- Hatami, S.; Hefler, J.; Freed, D.H. Inflammation and Oxidative Stress in the Context of Extracorporeal Cardiac and Pulmonary Support. Front. Immunol. 2022, 13, 831930. [Google Scholar] [CrossRef] [PubMed]
- García-de-la-Asunción, J.; Pastor, E.; Perez-Griera, J.; Belda, F.J.; Moreno, T.; García-del-Olmo, E.; Martí, F. Oxidative stress injury after on-pump cardiac surgery: Effects of aortic cross clamp time and type of surgery. Redox. Rep. 2013, 18, 193–199. [Google Scholar] [CrossRef] [PubMed]
- Murphy, D.A.; Hockings, L.E.; Andrews, R.K.; Aubron, C.; Gardiner, E.E.; Pellegrino, V.A.; Davis, A.K. Extracorporeal membrane oxygenation-hemostatic complications. Transfus. Med. Rev. 2015, 29, 90–101. [Google Scholar] [CrossRef]
- Wang, S.; Griffith, B.P.; Wu, Z.J. Device-Induced Hemostatic Disorders in Mechanically Assisted Circulation. Clin. Appl. Thromb. Hemost. 2021, 27, 1076029620982374. [Google Scholar] [CrossRef]
- Zwifelhofer, N.M.J.; Bercovitz, R.S.; Cole, R.; Yan, K.; Simpson, P.M.; Moroi, A.; Newman, P.J.; Niebler, R.A.; Scott, J.P.; Stuth, E.A.D.; et al. Platelet Function Changes during Neonatal Cardiopulmonary Bypass Surgery: Mechanistic Basis and Lack of Correlation with Excessive Bleeding. Thromb. Haemost. 2020, 120, 94–106. [Google Scholar] [CrossRef]
- Cherry, A.D. Mitochondrial Dysfunction in Cardiac Surgery. Anesthesiol. Clin. 2019, 37, 769–785. [Google Scholar] [CrossRef]
- Volk, L.E.; Mavroudis, C.D.; Ko, T.; Hallowell, T.; Delso, N.; Roberts, A.L.; Starr, J.; Landis, W.; Lin, Y.; Hefti, M.; et al. Increased cerebral mitochondrial dysfunction and reactive oxygen species with cardiopulmonary bypass. Eur. J. Cardiothorac. Surg. 2021, 59, 1256–1264. [Google Scholar] [CrossRef]
- Mazzeffi, M.; Lund, L.; Wallace, K.; Herrera, A.V.; Tanaka, K.; Odonkor, P.; Strauss, E.; Rock, P.; Fiskum, G. Effect of cardiopulmonary bypass on platelet mitochondrial respiration and correlation with aggregation and bleeding: A pilot study. Perfusion 2016, 31, 508–515. [Google Scholar] [CrossRef]
- Siewiera, K.; Labieniec-Watala, M.; Kassassir, H.; Wolska, N.; Polak, D.; Watala, C. Potential Role of Mitochondria as Modulators of Blood Platelet Activation and Reactivity in Diabetes and Effect of Metformin on Blood Platelet Bioenergetics and Platelet Activation. Int. J. Mol. Sci. 2022, 23, 3666. [Google Scholar] [CrossRef]
- Ehinger, J.K.; Piel, S.; Ford, R.; Karlsson, M.; Sjövall, F.; Frostner, E.; Morota, S.; Taylor, R.W.; Turnbull, D.M.; Cornell, C.; et al. Cell-permeable succinate prodrugs bypass mitochondrial complex I deficiency. Nat. Commun. 2016, 7, 12317. [Google Scholar] [CrossRef] [PubMed]
- Avram, V.F.; Chamkha, I.; Åsander-Frostner, E.; Ehinger, J.K.; Timar, R.Z.; Hansson, M.J.; Muntean, D.M.; Elmér, E. Cell-Permeable Succinate Rescues Mitochondrial Respiration in Cellular Models of Statin Toxicity. Int. J. Mol. Sci. 2021, 22, 424. [Google Scholar] [CrossRef] [PubMed]
- Bețiu, A.M.; Chamkha, I.; Gustafsson, E.; Meijer, E.; Avram, V.F.; Åsander Frostner, E.; Ehinger, J.K.; Petrescu, L.; Muntean, D.M.; Elmér, E. Cell-Permeable Succinate Rescues Mitochondrial Respiration in Cellular Models of Amiodarone Toxicity. Int. J. Mol. Sci. 2021, 22, 1786. [Google Scholar] [CrossRef] [PubMed]
- Sjövall, F.; Ehinger, J.K.; Marelsson, S.E.; Morota, S.; Frostner, E.A.; Uchino, H.; Lundgren, J.; Arnbjörnsson, E.; Hansson, M.J.; Fellman, V.; et al. Mitochondrial respiration in human viable platelets--methodology and influence of gender, age and storage. Mitochondrion 2013, 13, 7–14. [Google Scholar] [CrossRef]
- Gnaiger, E.; Kuznetsov, A.; Schneeberger, S.; Seiler, R.; Brandacher, G.; Steurer, W.; Margreiter, R. Mitochondria in the Cold; Springer: Berlin/Heidelberg, Germany, 2000; pp. 431–442. [Google Scholar]
- Avram, V.F.; Bîna, A.M.; Sima, A.; Aburel, O.M.; Sturza, A.; Burlacu, O.; Timar, R.Z.; Muntean, D.M.; Elmér, E.; Crețu, O.M. Improvement of Platelet Respiration by Cell-Permeable Succinate in Diabetic Patients Treated with Statins. Life 2021, 11, 288. [Google Scholar] [CrossRef]
- Landis, R.C.; Brown, J.R.; Fitzgerald, D.; Likosky, D.S.; Shore-Lesserson, L.; Baker, R.A.; Hammon, J.W. Attenuating the Systemic Inflammatory Response to Adult Cardiopulmonary Bypass: A Critical Review of the Evidence Base. J. Extra. Corpor. Technol. 2014, 46, 197–211. [Google Scholar]
- Zakkar, M.; Guida, G.; Suleiman, M.S.; Angelini, G.D. Cardiopulmonary bypass and oxidative stress. Oxid. Med. Cell. Longev. 2015, 2015, 189863. [Google Scholar] [CrossRef] [PubMed]
- Salameh, A.; Dhein, S. Strategies for Pharmacological Organoprotection during Extracorporeal Circulation Targeting Ischemia-Reperfusion Injury. Front. Pharmacol. 2015, 6, 296. [Google Scholar] [CrossRef]
- Mallet, R.T.; Olivencia-Yurvati, A.H.; Bünger, R. Pyruvate enhancement of cardiac performance: Cellular mechanisms and clinical application. Exp. Biol. Med. (Maywood) 2018, 243, 198–210. [Google Scholar] [CrossRef]
- Petrus, A.T.; Lighezan, D.L.; Danila, M.D.; Duicu, O.M.; Sturza, A.; Muntean, D.M.; Ionita, I. Assessment of platelet respiration as emerging biomarker of disease. Physiol. Res. 2019, 68, 347–363. [Google Scholar] [CrossRef]
- Sjövall, F.; Morota, S.; Hansson, M.J.; Friberg, H.; Gnaiger, E.; Elmér, E. Temporal increase of platelet mitochondrial respiration is negatively associated with clinical outcome in patients with sepsis. Crit. Care. 2010, 14, R214. [Google Scholar] [CrossRef]
- Ferguson, M.A.; Sutton, R.M.; Karlsson, M.; Sjövall, F.; Becker, L.B.; Berg, R.A.; Margulies, S.S.; Kilbaugh, T.J. Increased platelet mitochondrial respiration after cardiac arrest and resuscitation as a potential peripheral biosignature of cerebral bioenergetic dysfunction. J. Bioenerg. Biomembr. 2016, 48, 269–279. [Google Scholar] [CrossRef] [PubMed]
- Lelcu, T.; Bînă, A.M.; Dănilă, M.D.; Popoiu, C.M.; Aburel, O.M.; Arghirescu, S.T.; Borza, C.; Muntean, D.M. Assessment of Platelet Mitochondrial Respiration in a Pediatric Population: A Pilot Study in Healthy Children and Children with Acute Lymphoblastic Leukemia. Children 2021, 8, 1196. [Google Scholar] [CrossRef] [PubMed]
- Bînă, A.M.; Aburel, O.M.; Avram, V.F.; Lelcu, T.; Lința, A.V.; Chiriac, D.V.; Mocanu, A.G.; Bernad, E.; Borza, C.; Craina, M.L.; et al. Impairment of mitochondrial respiration in platelets and placentas: A pilot study in preeclamptic pregnancies. Mol. Cell. Biochem. 2022, 477, 1987–2000. [Google Scholar] [CrossRef] [PubMed]
- Pinti, M.V.; Fink, G.K.; Hathaway, Q.A.; Durr, A.J.; Kunovac, A.; Hollander, J.M. Mitochondrial dysfunction in type 2 diabetes mellitus: An organ-based analysis. Am. J. Physiol. Endocrinol. Metab. 2019, 316, e268–e285. [Google Scholar] [CrossRef]
- Schöttl, T.; Kappler, L.; Fromme, T.; Klingenspor, M. Limited OXPHOS capacity in white adipocytes is a hallmark of obesity in laboratory mice irrespective of the glucose tolerance status. Mol. Metab. 2015, 4, 631–642. [Google Scholar] [CrossRef]
- Winnica, D.; Corey, C.; Mullett, S.; Reynolds, M.; Hill, G.; Wendell, S.; Que, L.; Holguin, F.; Shiva, S. Bioenergetic Differences in the Airway Epithelium of Lean Versus Obese Asthmatics Are Driven by Nitric Oxide and Reflected in Circulating Platelets. Antioxid. Redox. Signal. 2019, 31, 673–686. [Google Scholar] [CrossRef]
- Tretter, L.; Patocs, A.; Chinopoulos, C. Succinate, an intermediate in metabolism, signal transduction, ROS, hypoxia, and tumorigenesis. Biochim. Biophys. Acta 2016, 1857, 1086–1101. [Google Scholar] [CrossRef]
- Piel, S.; Chamkha, I.; Dehlin, A.K.; Ehinger, J.K.; Sjövall, F.; Elmér, E.; Hansson, M.J. Cell-permeable succinate prodrugs rescue mitochondrial respiration in cellular models of acute acetaminophen overdose. PLoS ONE 2020, 15, e0231173. [Google Scholar] [CrossRef]
- Owiredu, S.; Ranganathan, A.; Eckmann, D.M.; Shofer, F.S.; Hardy, K.; Lambert, D.S.; Kelly, M.; Jang, D.H. Ex vivo use of cell-permeable succinate prodrug attenuates mitochondrial dysfunction in blood cells obtained from carbon monoxide-poisoned individuals. Am. J. Physiol. Cell Physiol. 2020, 319, C129–C135. [Google Scholar] [CrossRef]
- Bakare, A.B.; Rao, R.R.; Iyer, S. Cell-Permeable Succinate Increases Mitochondrial Membrane Potential and Glycolysis in Leigh Syndrome Patient Fibroblasts. Cells 2021, 10, 2255. [Google Scholar] [CrossRef] [PubMed]
Figure 1.
Routine respiration in the absence vs. presence of DMSO prior to (A) or after (B) cardiopulmonary bypass. Data are expressed as the mean ± SEM. ns = no statistical significance.
Figure 1.
Routine respiration in the absence vs. presence of DMSO prior to (A) or after (B) cardiopulmonary bypass. Data are expressed as the mean ± SEM. ns = no statistical significance.
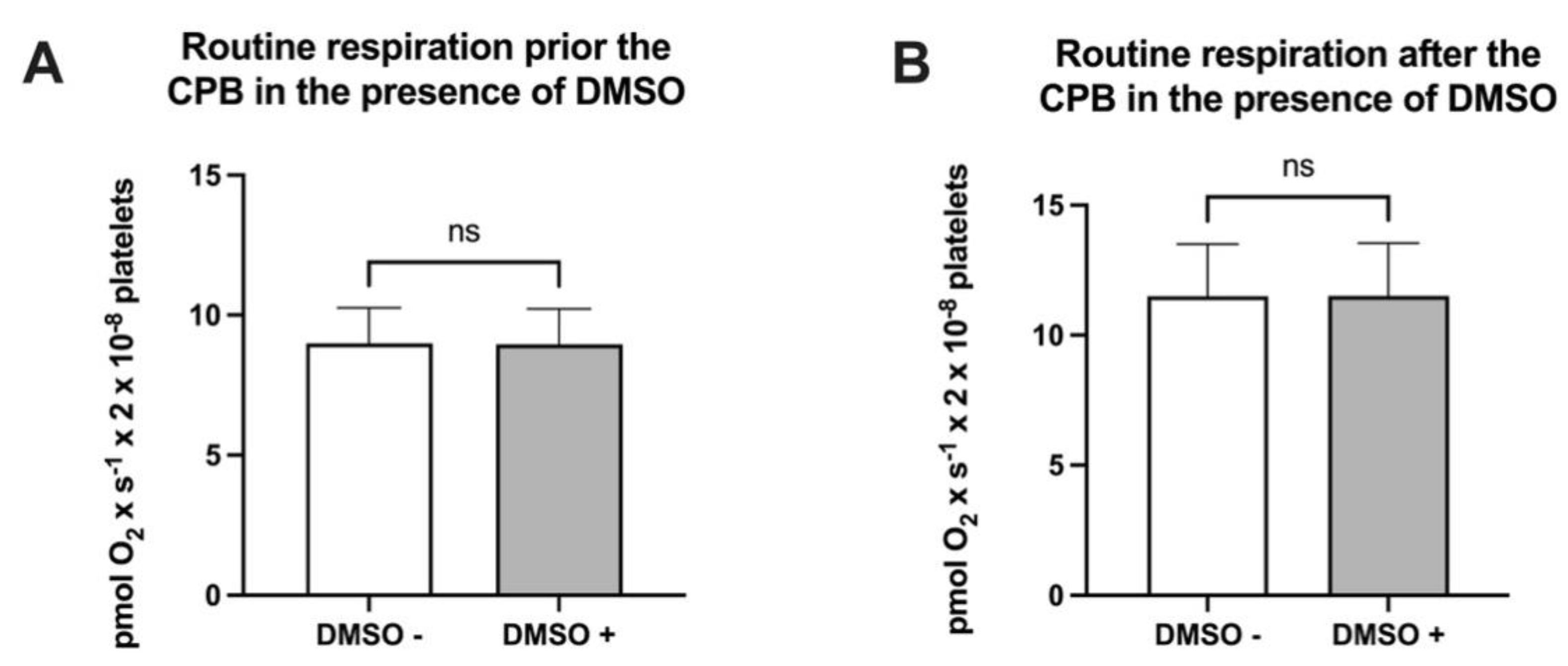
Figure 2.
Platelet mitochondrial respirometry prior to CBP in the absence vs. the presence of cell-permeable succinate NV118. (A) ROUTINE respiration, (B) LEAK respiration, (C) ET CAPACITY, (D) ETII CAPACITY. Data are expressed as the mean ± SEM of ROX-corrected respiration. * p < 0.5; **** p < 0.0001. ET capacity: electron transport capacity.
Figure 2.
Platelet mitochondrial respirometry prior to CBP in the absence vs. the presence of cell-permeable succinate NV118. (A) ROUTINE respiration, (B) LEAK respiration, (C) ET CAPACITY, (D) ETII CAPACITY. Data are expressed as the mean ± SEM of ROX-corrected respiration. * p < 0.5; **** p < 0.0001. ET capacity: electron transport capacity.
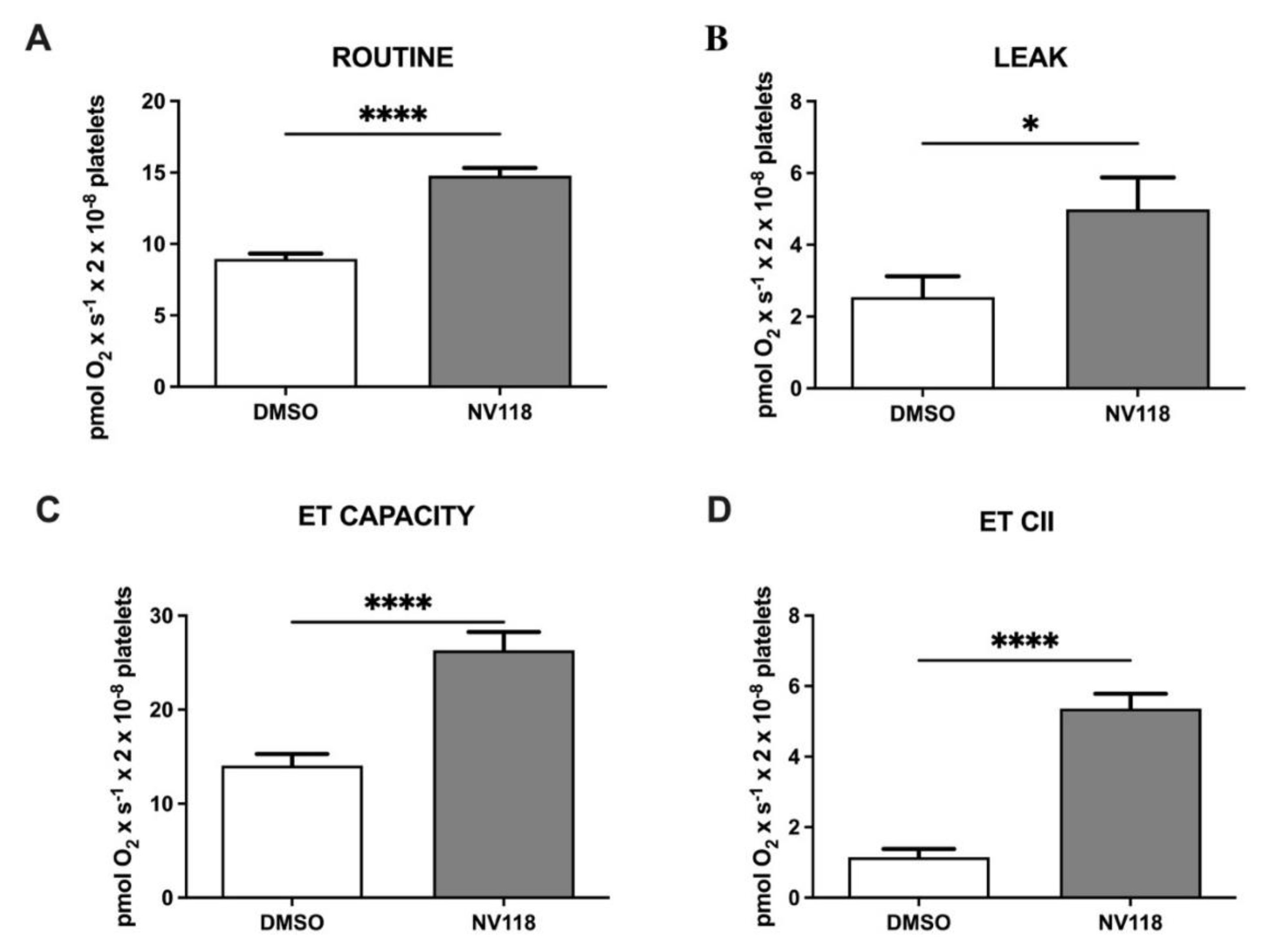
Figure 3.
Platelet mitochondrial respirometry after CPB in the absence vs. the presence the cell-permeable succinate NV118. (A) ROUTINE respiration, (B) LEAK respiration, (C) ET CAPACITY, (D) ETII CAPACITY. Data are expressed as the mean ± SEM. * p < 0.5; ** p < 0.01; *** p < 0.001; **** p < 0.0001. ET capacity: electron transport capacity.
Figure 3.
Platelet mitochondrial respirometry after CPB in the absence vs. the presence the cell-permeable succinate NV118. (A) ROUTINE respiration, (B) LEAK respiration, (C) ET CAPACITY, (D) ETII CAPACITY. Data are expressed as the mean ± SEM. * p < 0.5; ** p < 0.01; *** p < 0.001; **** p < 0.0001. ET capacity: electron transport capacity.
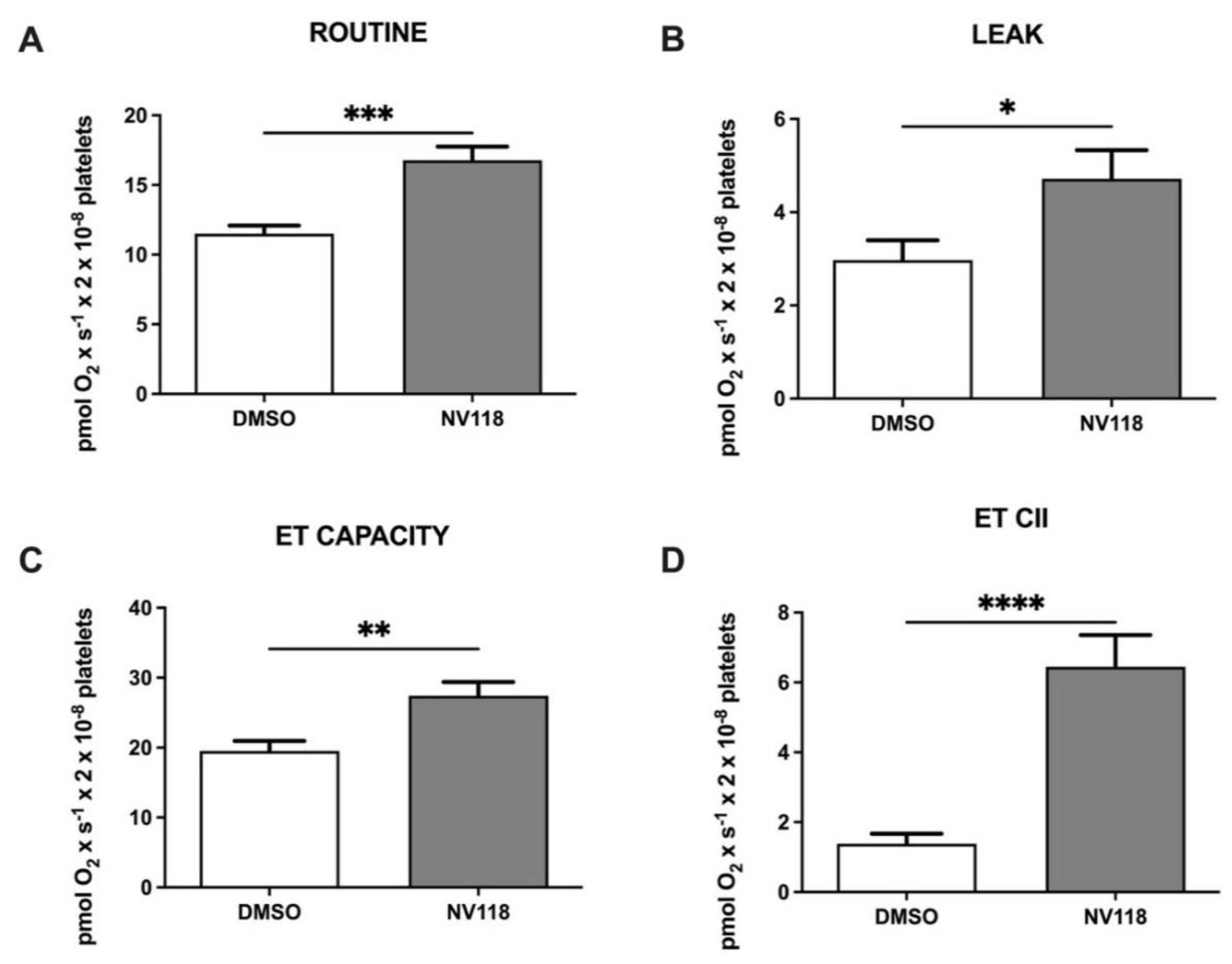
Figure 4.
Representative trace of HRR to assess the cell-permeable succinate effect on intact platelets after cardiopulmonary bypass. Red line (DMSO); green line (NV118).
Figure 4.
Representative trace of HRR to assess the cell-permeable succinate effect on intact platelets after cardiopulmonary bypass. Red line (DMSO); green line (NV118).
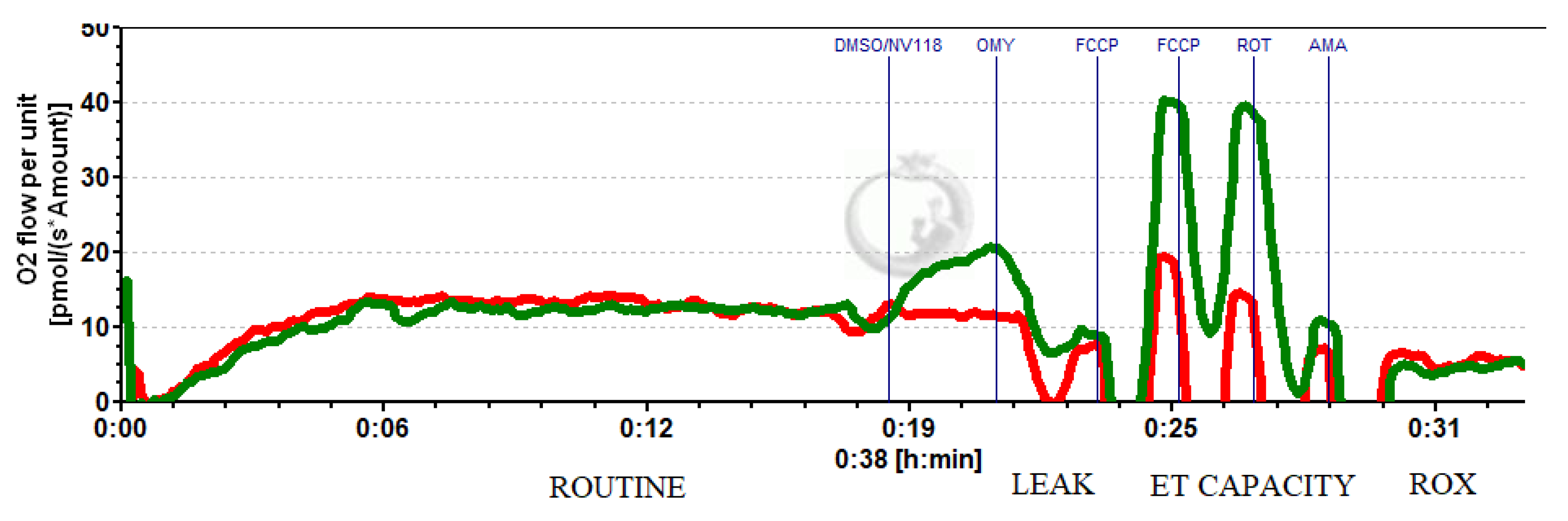
Table 1.
Characteristics of the study group.
PARAMETER | Value (mean ± SEM) |
---|---|
Age (y) | 64.6 ± 2.5 |
BMI (kg/m²) | 29.2 ± 1.3 |
Blood pressure (mmHg) | 131/74 ± 2.9/2.2 |
Heart rate (bpm) | 67 ± 2.76 |
RBC (mil/mm³) | 4.44 ± 0.12 |
Ht (%) | 40.93 ± 1.39 |
Hb (g/dL) | 13.42 ± 0.48 |
WBC (×10³/mm³) | 7.47 ± 0.56 |
PLT (×10³/mm³) | 239.6 ± 15.86 |
ESR (mm/h) | 31.6 ± 6.82 |
Creatinine (mg/dL) | 1.01 ± 0.1 |
Total cholesterol (mg/dL) | 192.1 ± 20.8 |
Blood glucose (mg/dL) | 115.2 ± 11.4 |
ASAT (U/L) | 24.7 ± 2.44 |
ALAT (U/L) | 33.8 ± 6.62 |
Serum sodium (mmol/L) | 141.7 ± 1.26 |
Serum potassium (mmol/L) | 4.15 ± 0.09 |
© 2022 Copyright by the authors. Licensed as an open access article using a CC BY 4.0 license.